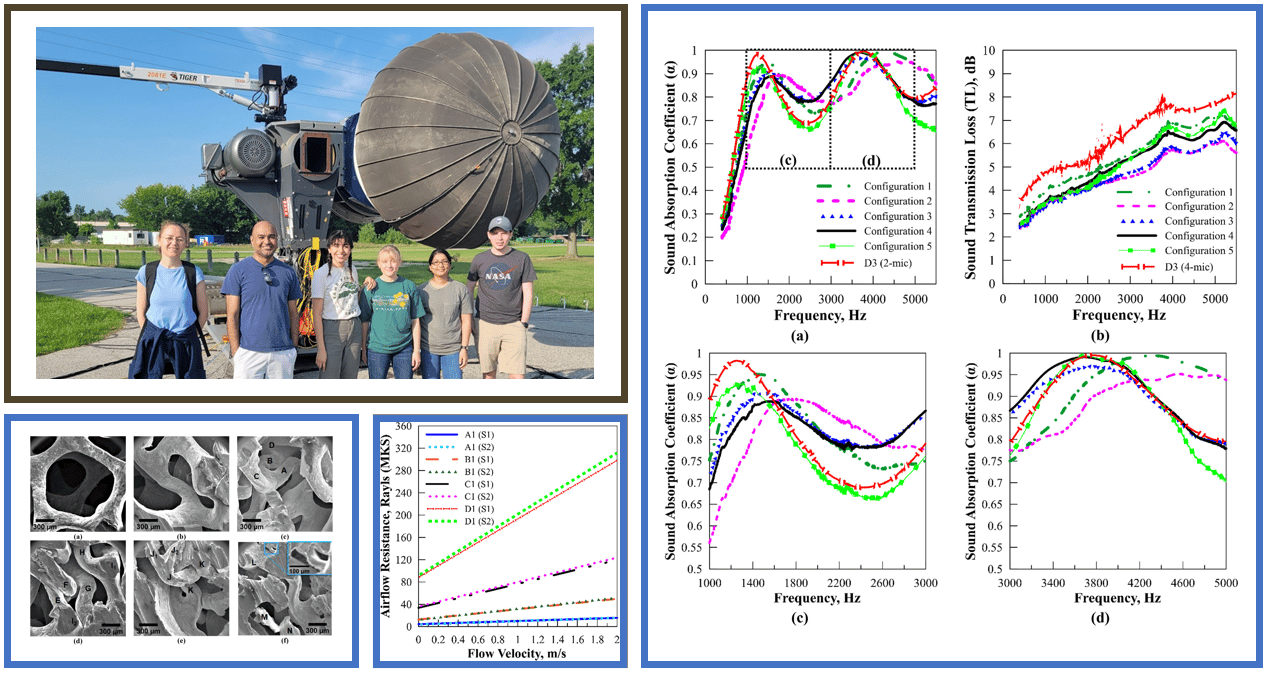
In this project, we are developing next-generation aircraft liners for broadband engine noise reduction using advanced cellular porous materials capable of withstanding extreme engine environments. Cellular materials offer a distinct advantage over traditional liner materials: their structural and functional properties are directly controlled by their local microstructural architecture. Thus, by engineering their microstructure, they can be tailored to provide significantly enhanced properties without parasitic mass addition. Here, our objective is to thoroughly characterize the effect of each individual microstructural parameter on the acoustic properties of open cell metal foams. The gained understanding will be leveraged to develop better predictive models for the design of foam metal liners and to inform the development of thin liners with variable through-thickness relative density.
Status: Current
Collaborators: Spirit Aerosystems, ERG Aerospace Corporation, NASA Langley, and NASA Glenn
Funding Agency: NASA, Spirit AeroSystems, Kansas Board of Regents
Architected Porous Sound Absorbers Enabled via 3D Printing
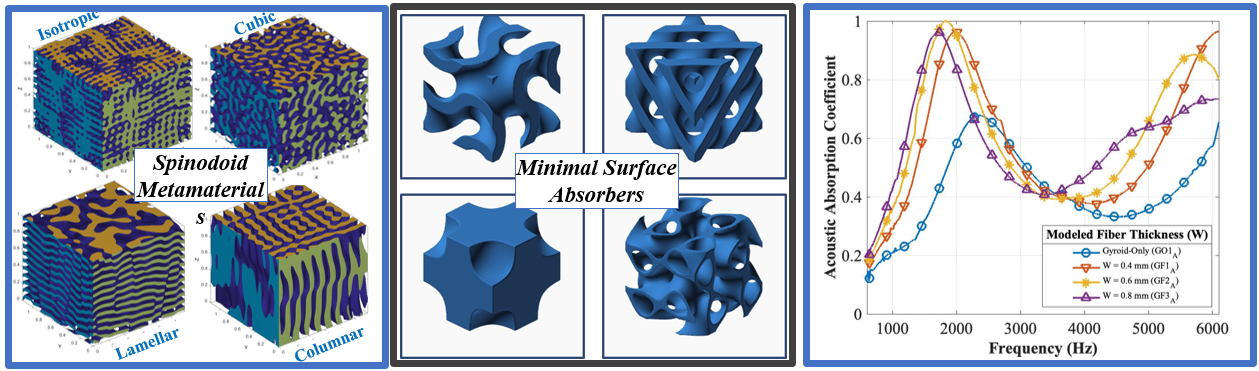
Current acoustical materials—such as foams and glass-fibers—provide good sound absorption and transmission loss characteristics; however, they suffer from three significant drawbacks: (1) their fabrication process limits material choices and inherently results in an anisotropic structure with significant batch-to-batch property variations; (2) their low mechanical stiffness prevents their use under load-bearing conditions; and (3) they cannot be used in high-pressure, high-temperature environments and suffer from poor flammability properties, releasing potentially toxic gases when inflamed. To overcome these issues, our research focuses on studying the acoustical behavior of architected porous structures that provide robust mechanical stiffness, can be fabricated using scalable advanced manufacturing techniques, and whose properties are directly controlled by their local microstructural architecture. Specifically, we focus on the design of novel pore geometries using recently developed implicit, field-based modeling tools and on elucidating their acoustical properties using analytical, computational, and experimental techniques.
Status: Current
Collaborators: Prof. J. S. Bolton (Purdue University), Dr. Tony Xue, Dr. Arash Rabbani, NASA Langley Research Center
Funding Agency: NASA, Lockheed Martin Space, Dell Inc.
Design and 3D Printing of Aerogel-based Ultra-lightweight Bulk Absorbers
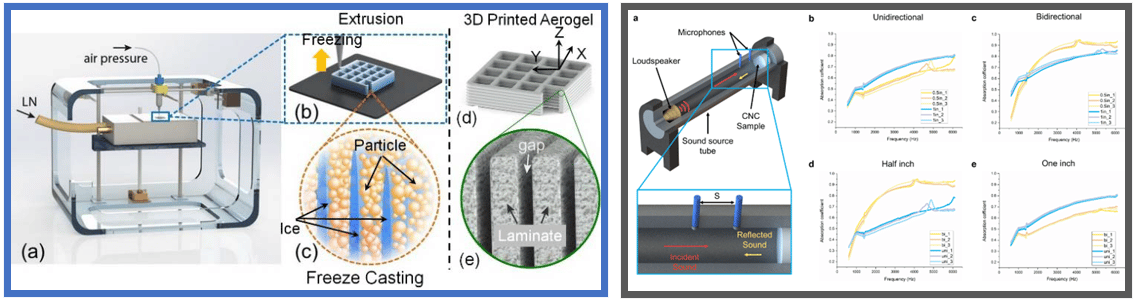
Aerogels are a class of synthetic materials that are derived by extracting the liquid component of a gel through supercritical drying and replacing it with gas. This process results in the formation of a nanoporous solid composed of up to 99.98% air by volume. Though aerogels have found applications in thermal blankets and as energy absorbers, their use in acoustic applications have been limited due to their fragility and lack of control over their macrostructure. Here, we utilize a novel 3D printing method, developed by Dr. Dong Lin, combining freeze casting and extrusion print silica aerogel bulk absorbers. Conventional processes such as freeze casting and sol-gel have been widely applied to manufacture aerogels; however, they are incapable of controlling macrostructures. Further, it is still significantly challenging to manipulate the aerogel microstructure during 3D printing; the current technologies can only manufacture compact structures or cross-sections with random pores. Here, 3D printing of silica with interconnected and aligned pores will be fulfilled by combining freeze casting with extrusion. This freeze casting process aligns the particles or other materials along the freezing direction. This process enables us to fabricate aerogel bulk absorbers with controlled microstructures with optimized sound absorption properties.
Status: Current
Collaborators: Prof. Dong Lin (Oregon State University)
Funding Agency: NASA, Kansas Board of Regents
Investigating the Acoustical Behavior of Granular Aerogel Agglomerates
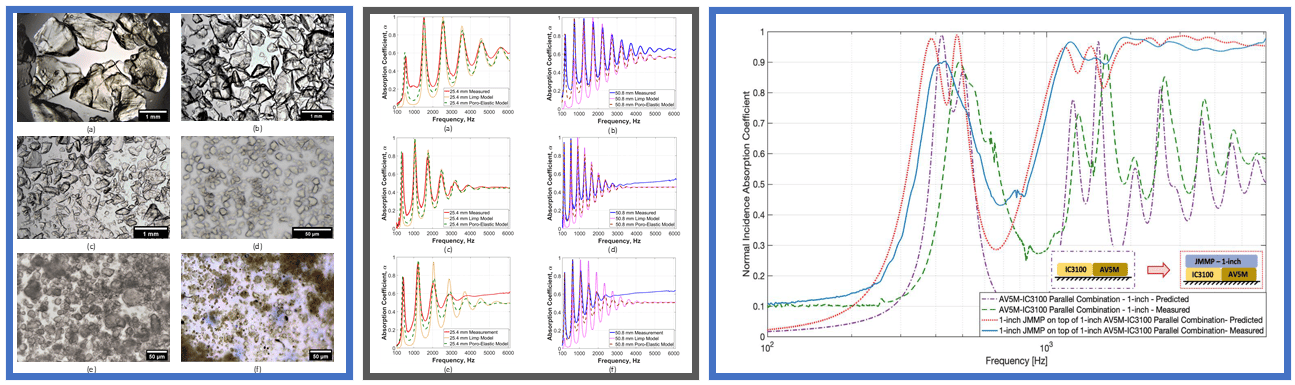
The attractiveness of aerogels for technological applications stems from their very low density—graphene aerogels are currently listed as the world’s least dense solids by Guinness World Records. Aerogels gain their novel properties from their underlying mesoporous structure, which results in a material with very low relative density, high surface area, high refractive index, very low thermal conductivity, and a low dielectric constant. While their thermal and optical properties have been extensively studied due to their potential aerospace and architectural insulation applications, their acoustical properties are less well understood. As part of our research, we are investigating the acoustical behavior of aerogel granules with the goal of enabling their use for ultralightweight noise reduction technologies. Thus far, our work has shown that there exists a strong correlation between their particle size and sound absorption behavior and reveal important differences between the absorption trends of granular aerogel particles larger than 100 μm and those smaller than 50 μm. Further, our results show that while agglomerates of larger aerogel granules can be modeled as limp porous media, smaller aerogel granules require a poro-elastic formulation. Recently, we have also demonstrated the potential of incorporating such granules within a layered medium to create broadband noise reduction packages.
Status: Current
Collaborators: Prof. J. S. Bolton (Purdue University), Dr. Tony Xue (Midea Corporation), JIOS Corporation.
Funding Agency: NASA, Kansas Board of Regents
Design and 3D Printing of Aerogel-based Ultra-lightweight Bulk Absorbers
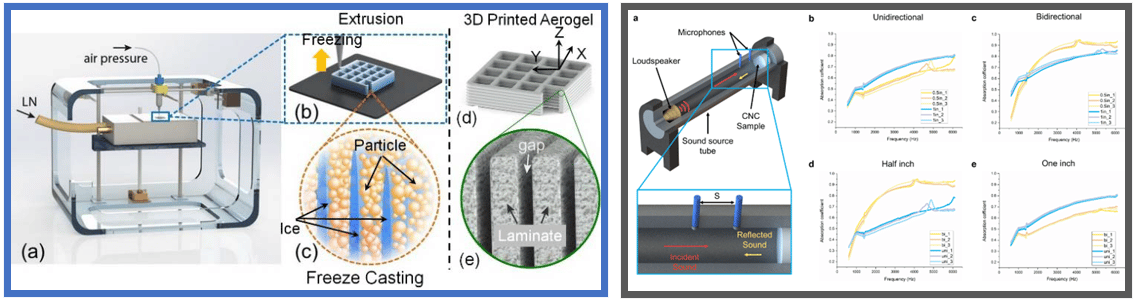
Aerogels are a class of synthetic materials that are derived by extracting the liquid component of a gel through supercritical drying and replacing it with gas. This process results in the formation of a nanoporous solid composed of up to 99.98% air by volume. Though aerogels have found applications in thermal blankets and as energy absorbers, their use in acoustic applications have been limited due to their fragility and lack of control over their macrostructure. Here, we utilize a novel 3D printing method, developed by Dr. Dong Lin, combining freeze casting and extrusion print silica aerogel bulk absorbers. Conventional processes such as freeze casting and sol-gel have been widely applied to manufacture aerogels; however, they are incapable of controlling macrostructures. Further, it is still significantly challenging to manipulate the aerogel microstructure during 3D printing; the current technologies can only manufacture compact structures or cross-sections with random pores. Here, 3D printing of silica with interconnected and aligned pores will be fulfilled by combining freeze casting with extrusion. This freeze casting process aligns the particles or other materials along the freezing direction. This process enables us to fabricate aerogel bulk absorbers with controlled microstructures with optimized sound absorption properties.
Status: Current
Collaborators: Prof. Dong Lin (Kansas State University) and Aerogel Technologies LLC.
Funding Agency: NASA, Kansas Board of Regents
Surrogate Modeling of Sound Absorbers with Through-Thickness Property Gradients
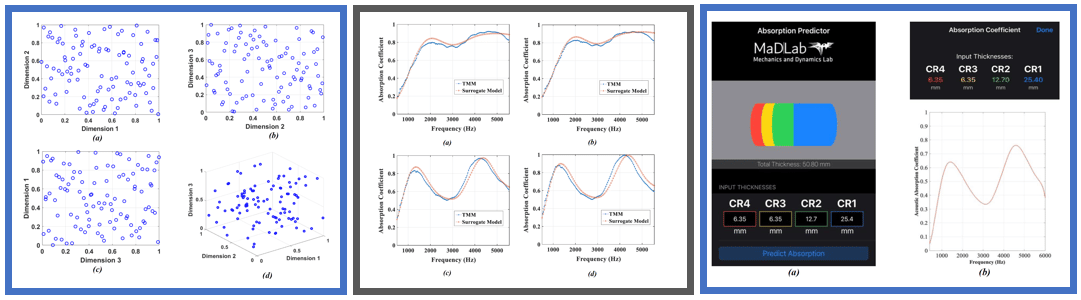
Engineering problem solving often requires extensive lab experimentation, which usually entails substantial time and monetary investments. Surrogate modeling, or metamodeling, offers an alternative approach. Often described as ‘model of a model’, surrogate or metamodels are low-fidelity machine learning models that can be trained using finite measurements to rapidly provide solutions at intermediary locations. In this work, we are developing Kriging-based surrogate models to predict the sound absorption performance of porous foams with user-defined density gradients. We use experimentally validated transfer matrix models to generate the necessary training data and then deploy a spatial analysis method to create influence surfaces around each sample data point and depict its effect on the observed output by using Kriging’s basis functions. The surrogate model has an in-built machine learning framework that uses a genetic algorithm and a maximum likelihood function to tune the weights that define these influence surfaces. Using this approach, we have successfully developed a data-fed, supervised prediction model that estimates absorption coefficient of a virtual metal foam sample. We have also implemented the developed metamodel as an iOS application as a potential low-cost tool to reduce testing costs and enable rapid design iterations for acoustic design problems.
Status: Current
Collaborators: NASA Glenn Research Center, Dr. Mai Dao (WSU)
Acoustic metamaterials with negative mass and modulus behavior
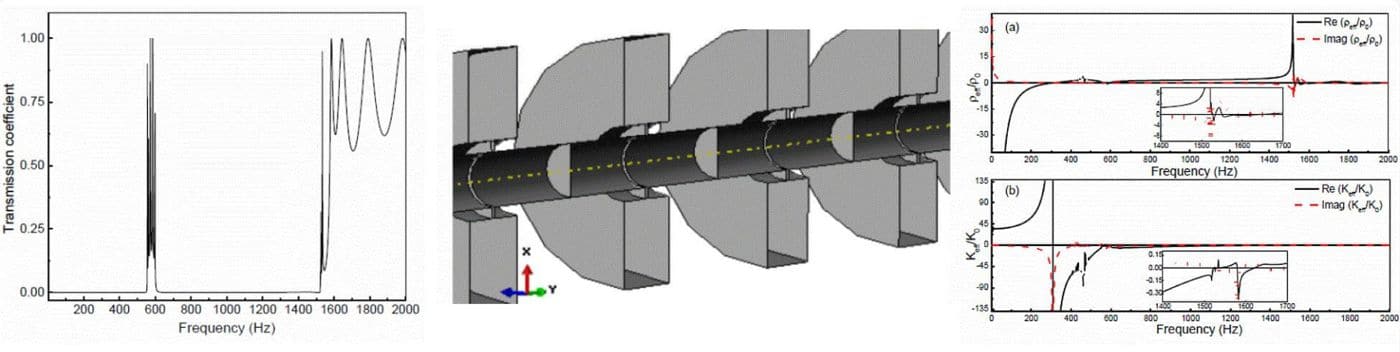
We demonstrate a negative bulk modulus metamaterial based on the concept of expansion chambers. Addition of a neck region to an ordinary expansion chamber significantly improves its transmission loss characteristics at low frequencies and the resulting structure displays a negative bulk modulus behavior. Additionally, membrane based metamaterials are analyzed. Using FEM, the negative density behavior of a membrane carrying a center mass and of a tensioned membrane array is analyzed and the inherent similarity of the two designs is discussed. Further, the modified expansion chamber is combined with an array of stretched membranes and the resulting structure is analyzed for double negative behavior.
Status: Completed
Collaborators: Prof. CT Sun (Purdue University)
Funding Agency: Office of Naval Research